Experiments
Here are experiments our science specialists have selected to support the IB* topic.
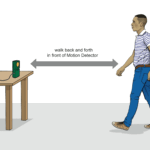
Graph Matching
Experiment #1 from Physics with Vernier
- Analyze the motion of a student walking across the room.
- Predict, sketch, and test position vs. time kinematics graphs.
- Predict, sketch, and test velocity vs. time kinematics graphs.
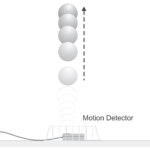
Ball Toss
Experiment #6 from Physics with Vernier
- Collect position, velocity, and acceleration data as a ball travels straight up and down.
- Analyze position vs. time, velocity vs. time, and acceleration vs. time graphs.
- Determine the best-fit equations for the position vs. time and velocity vs. time graphs.
- Determine the mean acceleration from the acceleration vs. time graph.
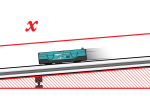
Determining g on an Incline
Experiment #4 from Physics with Vernier
- Use a Motion Encoder System to measure the velocity and acceleration of a cart rolling down an incline.
- Determine the mathematical relationship between the angle of an incline and the acceleration of a cart rolling down the incline.
- Determine the value of free fall acceleration, g, by using an extrapolation on the acceleration vs. sine of track angle graph.
- Determine if an extrapolation of the acceleration vs. sine of track angle is valid.
- Educational Standard
- International Baccalaureate (IB)
- Subject
- Physics
- Section
- Core
- Topic
- 2. Mechanics
* The IB Diploma Program is an official program of the International Baccalaureate Organization (IBO) which authorizes schools to offer it. The material available here has been developed independently of the IBO and is not endorsed by it.